The New Transformers
BU's College of Engineering leading the way in synthetic biology innovation
Boston University College of Engineering (ENG) Assistant Professor Wilson Wong (biomedical engineering) is attempting to develop the next generation of personalized smart cancer therapy by taking a cancer patient’s immune system cells and installing novel genetic programs to control when, where, and how strongly the engineered cells should respond to cancer cells. To rapidly and predictably engineer desired properties in human immune cells to treat leukemia and other cancers, Wong is applying sophisticated techniques from a rapidly growing and highly promising field that was pioneered at the College of Engineering: synthetic biology.
Synthetic biology brings together engineers, molecular biologists, physicists, and chemists who apply engineering principles to conceive, design, and build molecular biological systems that effectively rewire and reprogram cells, microbes, and other living organisms to perform specified tasks. They achieve this by using molecular biology and computational tools to make strategic modifications to genetic “circuits,” networks, and pathways that perform logical functions akin to those observed in electrical circuits. Through such modifications, synthetic biologists promise to improve both our understanding of the inner workings of life and the quality of our lives as they advance novel solutions to critical challenges in health care, energy, the environment, global security, food production and safety, and other domains.
ENG and Synthetic Biology: Right There from the Start
Since the early 1960s, scientists and engineers have sought to engineer microorganisms to perform tasks that address such challenges. It wasn’t until the 1990s, however, that the enabling technology would emerge with major advances in genomic sequencing and the rise of systems biology, which combines experimental techniques with computational approaches to reverse engineer cellular networks and pathways. By the end of the decade, researchers who recognized the cellular network as a hierarchy of modular molecular components—proteins, genes, and other bits of DNA—began to tune and rearrange these components, thereby changing the behavior of the network as a whole. Rather than reverse engineering the genome, they attempted to forward engineer it to enable living organisms to perform a variety of functions.
One of the first two attempts to perform such forward-engineering took place at Boston University in 1999, when Professor James J. Collins (biomedical engineering, materials science and engineering, systems engineering) and his biomedical engineering graduate student Timothy Gardner (ENG’00) tweaked E. coli bacterial genes so they could be induced to either make or not make certain proteins. This two-gene on/off switch for a biological circuit—together with a similar breakthrough at Princeton University around the same time—were ultimately hailed in the journal Nature as the “defining pair of experiments” marking the birth of synthetic biology.
Since then, the field dramatically expanded its capabilities as practitioners developed more complex biological circuits and an increasingly diverse array of well-characterized genetic components from which to assemble them. These developments, coupled with significant gains in the past five years in computational power and DNA assembly capabilities, have accelerated efforts to re-engineer living organisms to produce clean energy, kill cancer cells, remediate oil spills, detect weapons, and perform other tasks that move society forward.
ENG Emerges as a Leader in Synthetic Biology
Carving out renovated space at 36 Cummington Mall, ENG created a world-class facility to provide a platform for synthetic biology innovation and train its next generation of researchers. This platform serves ENG synthetic biology core faculty assistant professors Douglas Densmore (electrical and computer engineering, biomedical engineering, bioinformatics), Ahmad (Mo) Khalil (biomedical engineering, bioinformatics) and Wilson Wong (biomedical engineering). Together, they are reinforcing BU’s place among the preeminent synthetic biology institutions in the nation.
Drawing on non-biology backgrounds emphasizing electrical engineering (Densmore), mechanical engineering (Khalil), and chemical engineering (Wong), the core faculty are joined by 11 associate faculty members from BU’s College of Engineering, College of Arts & Sciences, and School of Medicine whose work relates to synthetic and systems biology.
“A central goal of our synthetic biology faculty will be to prepare the next generation of synthetic biologists for this multidisciplinary type of research at an early stage, and to challenge them to think conceptually and creatively about how engineering can help in understanding life,” says Khalil.
The facility—slated for eventual relocation to a new Center for Integrated Life Science & Engineering building nearby—includes newly renovated wet and dry labs and computational space. To advance its research agenda, the faculty plan to develop and support large-scale, collaborative projects, and organize a seminar series and annual symposium on synthetic biology. The faculty will also support research training, education, and outreach activities to ensure that students of all levels can learn about the fundamentals and practice of synthetic biology.
As the core faculty members advance tools and techniques to create genetic parts and circuits to reprogram bacterial and mammalian cells more efficiently, cheaply, and precisely, they are also applying these approaches to better understand biological systems and improve the quality of life, with a primary focus on medical diagnostics and treatments.
Wilson Wong: Engineering Smart Cancer Therapies
Wilson Wong aims to engineer “smart” therapeutic agents that can adapt to different patients and eradicate a wide range of cancer cells while avoiding side effects.Formerly a postdoctoral scholar in cellular and molecular pharmacology at UC-San Francisco, Wilson Wong joined the BU faculty in 2012 and a year later won the National Institutes of Health Director’s New Innovator Award, which provides up to $1.5 million in research funding for five years. The award supports exceptionally creative, early-career researchers pursuing highly innovative projects with the potential to transform their field of endeavor and bring about improved health outcomes.
One major challenge in developing a curative therapy like this one is that a tumor is composed of diverse sets of cancerous and noncancerous cells.
Wong’s research on personalized smart cancer therapy more than qualifies. He is currently working to engineer genetic circuits to improve the efficacy and safety of adoptive T cell therapy, a treatment for leukemia and related blood cancers—and potentially other tumors—in which a patient’s immune system is reprogrammed. This entails removing millions of a patient’s T cells (a kind of white blood cell) and inserting new genes that make it possible for the T cells to kill cancer cells. When the modified T cells are returned to the patient’s veins, they ideally kill the cancer.
One major challenge in developing a curative therapy like this one is that a tumor is composed of diverse sets of cancerous and noncancerous cells expressing different biomarkers at varying levels—within and among patients. Current therapeutic agents that target specific cellular processes or signaling pathways are not effective against such diverse cell populations, and those that target cancer-specific mutations or markers miss many of the different cancerous cells found in a tumor. In addition, drugs that are effective for some patients may be ineffective or have severe side effects for others. So Wong aims to engineer “smart” therapeutic agents that can adapt to different patients and eradicate a wide range of tumor cells while avoiding systematic side effects.
“We will leverage our expertise in synthetic gene circuits, T cell engineering, and computational modeling to develop novel gene networks in T cells that control when, where, and which cancer-specific receptors are being expressed, thus reprogramming the spatial and temporal activity of cancer-killing T cells,” he explains.
The resulting engineered T cells would be the most sophisticated therapeutic agents ever developed, providing an unprecedented level of flexibility, precision, and personalization. The genetic circuits and design principles developed through this research could serve as a general platform that could be applied and have immediate and broad impact on many cancers. “By bringing two emerging fields of science—cell-based immunotherapy and synthetic biology—to cancer research, we hope to fundamentally change cancer treatment,” says Wong.
Below: a. A tumor is composed of diverse sets of cancerous and noncancerous cells expressing different biomarkers at varying levels, and tumors from different patients are also very different. One reason why many cancer therapies ultimately fail is that current therapeutic agents were never designed to combat such diversity. b. In adoptive immunotherapy, a tumor-specific receptor is introduced to patients’ T cells, enabling the T cells to find tumors and destroy them. A tumor-specific receptor called chimeric antigen receptor (CAR) has shown phenomenal clinical results in treating leukemia. c. Wong aims to engineer smart cancer therapies that kill a wide variety of cancer cell types without introducing side effects. He will apply synthetic biology technologies to develop genetic circuits that can control when, where, and how the T cells are activated.
Douglas Densmore: Automating Synthetic Biology
Douglas Densmore’s work focuses on automating processes to increase efficiency, remove errors, and improve reproducibility. Liquid handling robots and microfluidic devices are part of this ecosystem.Three years before joining BU’s faculty in 2010 and while completing his PhD in electrical engineering at UC-Berkeley, Douglas Densmore was surprised that genetic circuits presented in a talk by a bioengineering expert were described much like electrical circuits. Densmore has been working ever since to streamline the process of producing novel genetic circuits by extending the analogy to their design process. He may be the only person in the world whose entire research agenda is devoted to automating synthetic biology, moving it from an art form to a more rigorous, standardized discipline.
His elevator speech boils down to this: “I make the specification, design, and physical assembly of synthetic biology systems easier with the use of computational tools.” Specifying a synthetic biology system is akin to laying out a blueprint for a new building, he says, one that’s comprehensive and detailed enough so that there’s no ambiguity about what the final structure should look like. Designing a specified system entails transforming that blueprint into the biological components, or parts, needed to build it. The final step—assembly—is done not by hand, but with robots or microfluidic chips that move liquids and DNA around.
Densmore developed one software tool for each stage of the process, complete with a colorful name: Eugene for specification, Clotho for design, and Puppeteerfor assembly. Since joining the BU faculty, he has upgraded these technologies while creating seven additional specification, design, and assembly tools.
Funded by a $1.1 million grant from the National Science Foundation, Densmore is now working to transform Clotho into commercially viable software via a University spinoff called Lattice Automation. Meanwhile, he is beginning to fuse his original and current fields, electrical engineering and synthetic biology, with a long-term focus on hybrid bioelectronics.
His elevator speech boils down to this: “I make the specification, design, and physical assembly of synthetic biology systems easier with the use of computational tools.”
“My goal is to develop several simple biological parts and package them in electronics,” Densmore explains. “The hybrid systems would be built automatically with software.”
For example, a bacterium might be engineered to react to light by producing a change in the pH of a liquid in a microfluidic chamber. Electronics surrounding the chamber would sense the pH change and tweet a message indicating that the bacteria has detected light. One might place the bioelectronics device inside concrete pillars holding up a bridge, where it could report cracks at the first sign of light.
“People have done this before, but our hook will be to combine synthetic biology-designed organisms with electronics using software tools,” says Densmore. “For this to work on an industrial scale, the systems we make will have to be cheap enough to throw away and operate autonomously for at least 24 hours, which is about how long bacteria can survive.
Toward that end, Densmore and Khalil are working to combine microfluidics and synthetic biology approaches to construct a programmable, bioelectronic device that could be used in bioremediation, biosensing, and other applications.
Dashboard for Clotho, a tool developed by Douglas Densmore to design synthetic biology systems. Funded by a $1.1 million grant from the National Science Foundation, Densmore is now working to transform Clotho into commercially viable software.Ahmad (Mo) Khalil: New Parts and Pathways to Reprogram Cells
Ahmad (Mo) Khalil’s lab focuses on advancing synthetic biology approaches to provide a better understanding of the design principles of complex biological systems, and to engineer cells for useful applications in medicine, energy, and the environment.A former postdoctoral fellow in Collins’ lab and BU faculty member since 2012, Mo Khalil became interested in synthetic biology as a PhD student at MIT. Named one of the 20 most promising early-career genomics researchers around the world in 2013 by GenomeWeb, Khalil has developed a new, reliable, and expanded toolkit of genetic circuit components and microfluidic devices that synthetic biologists can use to program novel behaviors in living cells.
For example, working with colleagues at BU, Harvard Medical School, Massachusetts General Hospital, and MIT, he has advanced a new method for constructing and analyzing genetic circuits in eukaryotes—organisms whose cells contain nuclei. Instead of constructing these circuits with off-the-shelf parts from bacteria and porting them into eukaryotes, as most synthetic biologists do, Khalil and his collaborators have engineered these circuits using modular, functional parts from the eukaryotes themselves. Potential applications range from stem cell therapeutics to in-cell diagnosis of cancer and other diseases.
In addition to advancing the state of the art of synthetic biology, Khalil is applying its techniques to improve our understanding of biological organisms and devise new ways to treat disease.
With the support of a National Science Foundation CAREER award in 2014, he is working to better understand the mechanisms underlying how organisms adapt to changing environments, a classic problem in evolutionary biology. He’s testing a theory that prions—proteins that can cause neurodegenerative diseases in mammals—equip microbes with an enhanced capability to survive under fluctuating environmental conditions.
“This work will have broad implications for our basic understanding of evolution and development, and could provide us with interesting new strategies for engineering and evolving cellular systems for application,” says Khalil.
In another project that promises to directly improve the effectiveness of health care, Khalil is helping to spearhead a method that could accelerate diagnosis and treatment of bacterial infections, a growing public health problem.
“Our goal is to create a universal diagnostic platform that can perform antibiotic susceptibility testing across a full spectrum of antibiotics and to do this as rapidly as possible.”
Pinpointing the most effective treatment for a bacterial infection can take several days as clinicians culture bacteria and select the most effective antibiotic. Meanwhile, patients receive broad-spectrum antibiotics that could be far less effective, leaving them prone to spreading the infection and generating antibiotic-resistant bacteria. But a new, culture-free diagnostic approach that Khalil and Collins are exploring could identify the most suitable antibiotic in just a few hours, leading to rapid treatment and infection containment. The researchers are developing systems to detect early cellular changes that occur in susceptible bacterial cells when treated with antibiotics.
“Our goal is to create a universal diagnostic platform that can perform antibiotic susceptibility testing across a full spectrum of antibiotics,” says Khalil. “And to do this as rapidly as possible.”
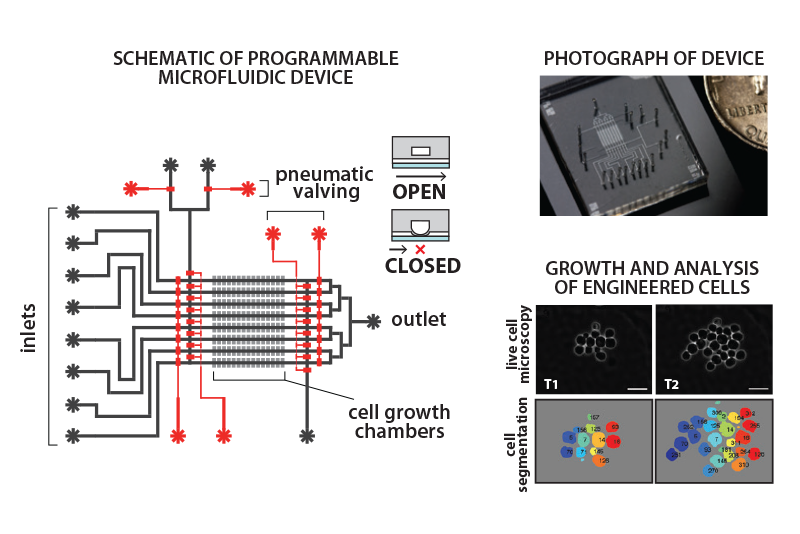
The Future of Synthetic Biology
In the coming decades, synthetic biology is likely to generate many more new insights about the genetic circuitry and behavior of biological organisms and sophisticated devices that exploit that circuitry to diagnose and treat disease, produce renewable biofuels, and meet other societal challenges. The wellspring of innovation in the field will be in today’s living organisms, which embody a “knowledgebase” of genetic circuits, networks, and pathways that have evolved over countless millennia to perform complex functions in a wide range of environments.
Leading the way in translating information from life’s knowledgebase into synthetic biology techniques and applications from the very beginning, the College of Engineering is poised to continue shaping the field and advancing life-enhancing solutions for many years to come.
This article from below link:
https://www.bu.edu/articles/2014/the-new-transformers/